While people on Earth are thinking about self-driving cars, Christopher “Chrispy” Petersen is focused on self-driving satellites.
“A satellite that nudges itself in the right direction or performs some aspect of self-repair lets people in the control room focus on other, important mission-oriented tasks,” he says.
Petersen, an assistant professor of aerospace and mechanical engineering, loves pushing the limits of satellite capabilities.
“If we already have all this real-time data from sensors and fine manipulation via actuators, can we use them in a novel way? Can we chain together our algorithms to do the mission in a unique and unconventional way?” he asks.
That pioneering mindset comes naturally to Petersen and other UF space researchers. It’s led them to forge paths in brain research, muscle atrophy and space manufacturing. Persistence and bootstrapping also help. As a prime example, Petersen used Lego robots early in his career to do crucial satellite simulations on a shoestring budget.
Rachael Seidler has spent years studying spaceflight’s effects on the human brain and body. It started with a random but timely email nearly three decades ago.
Seidler was a graduate student at Arizona State University in the mid-1990s, researching sensory conflict that leads to motion sickness and other problems. One day, she learned that NASA offered graduate student fellowships.
It was the ultimate cold pitch, reaching out to a scientist at the Johnson Space Center in Houston. Seidler didn’t know the researcher but she had some ideas. He agreed to a chat.
“I got the fellowship and collaborated with the same person and same team in his lab for 25 years. It ended up just being a great connection with an awesome mentor,” Seidler says.
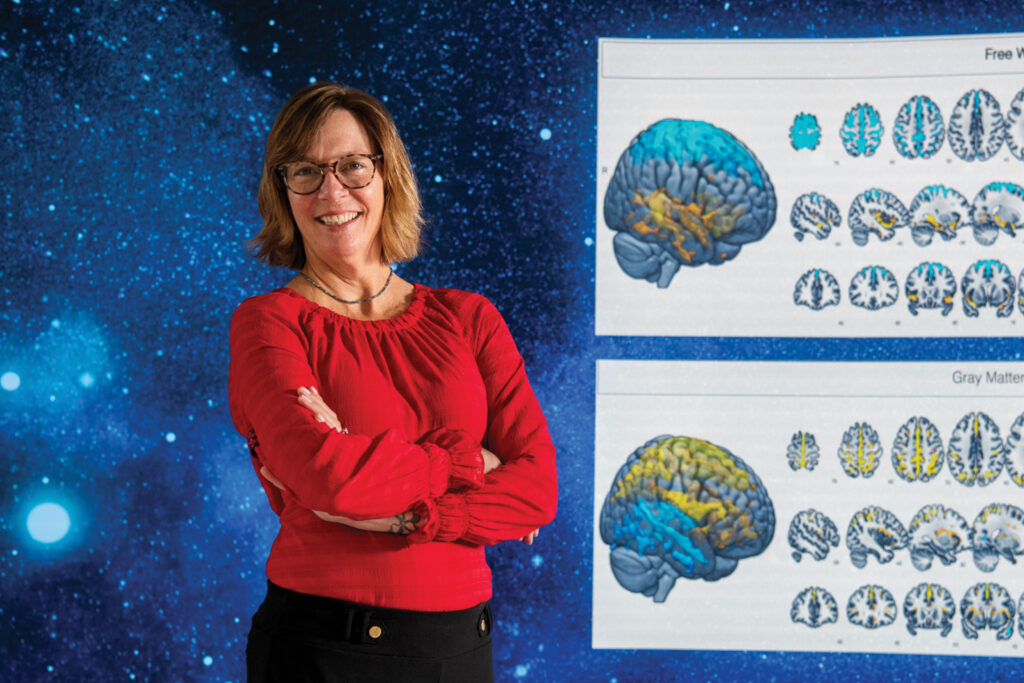
After postdoctoral work in Minnesota and a professorship in Michigan, she joined UF as a professor of applied physiology and kinesiology in 2017.
Even now, Seidler admits that the factual and historical minutiae of space flight don’t hold much interest. For her, it’s an “incredible opportunity” to study neuroplasticity — the brain’s ability to adapt to changes and experiences. It’s also a way to better understand and help counteract the many physical effects of space travel and microgravity.
“A lot of our physiology and anatomy has evolved to either work with gravity or against it. I’m always interested in basic science questions but realize I have to fit that into helping NASA solve very applied problems,” she says.
As lengthy missions become more common and space agencies consider sending humans to Mars, Seidler works at understanding and mitigating the health hazards of spaceflight, especially those caused by microgravity.
In microgravity, body fluids shift toward the head. The brain also moves upwards, pressing against the top of the skull. That affects the part of the brain that controls movement of the lower body. Meanwhile, the lower body muscles aren’t used for standing and walking in microgravity because astronauts are floating.
Work by Seidler and others has shown that fluid-filled spaces in the middle of the brain known as ventricles expand in space, potentially contributing to a diminished vision condition known as Spaceflight Associated Neuro-ocular Syndrome. More study is needed to determine how shifts in body fluid and brain position affect astronauts’ health and performance, Seidler says.
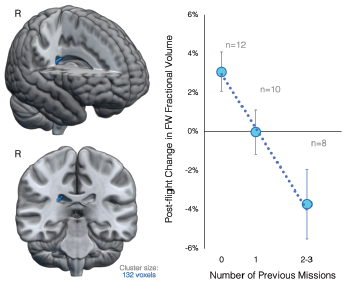
She has also shown how astronauts who are adapting to low gravity undergo brain changes that resemble accelerated aging. Most of those changes involve degraded white matter in the brain, which is crucial for quick and efficient brain signaling. Astronauts with more affected white matter have the most problems with balance and mobility after returning to Earth, Seidler has found.
That also underscores another challenge she is working to unravel: Astronauts all respond differently to the rigors of space. Some of them might have significant motion sickness while others have none. Some astronauts struggle to stand up and walk a straight line when they first return to Earth while others don’t.
To better understand those effects, Seidler recently started a NASA-funded study tracking space crews for five years after their flights. An array of brain scans, behavioral measures and eye evaluations are meant to reveal brain and eye changes and the long-term health effects of space travel.
For Seidler, helping astronauts be safe and highly functional in space is important. So, too, is more knowledge of the brain’s resilience. A fuller understanding of expanding brain ventricles in astronauts could lead to better treatments for a similar but naturally occurring disorder in the population at large, she says.
More broadly, Seidler says microgravity is a good analog for dealing with medical problems on Earth. The inner ear balance sensor is impacted not just by microgravity but also by normal aging, tumors and other conditions.
“Truly understanding how we compensate when one or more sensory systems are dysfunctional and looking for ways to accelerate that compensation can be enormously beneficial for people on Earth,” she says.
Over the years, Seidler has learned what really drives her — and it’s not just solving the problems of spaceflight.
“I think it’s really important to understand the potential boundaries for adaptation and neuroplasticity in the healthy brain,” she says.
Satellite Precision
Ask Chrispy Petersen about controlling and optimizing satellites and he gives a simple but powerful example.
“You can’t put a laptop into space,” Petersen says.
For Petersen, a typical computer’s limitations reveal the many difficulties that come with keeping satellites on track and bringing them into proximity. He ticks off some of the issues: Radiation in space would fry a laptop within six months. Computer processors on satellites are a decade behind the ones on Earth. Even simply having enough electrical power to complete complex, automated tasks in orbit is an issue.
To solve these challenges, his lab optimizes satellite operations, hardware and software amid extreme mission and safety constraints. That means docking a satellite while minimizing fuel use, coaxing a satellite into a particular orbit as quickly as possible or autonomously exploring unknown regions around the moon. Bringing multiple satellites close together to complete these goals is a big complication.
Ultimately, Petersen says, those solutions involve a mix of speed, safety and reliability — all of which needs to be optimized for peak performance. Much of the work in his Spacecraft Technology and Research Laboratory focuses on docking or operating satellites in close quarters — about 300 miles apart. To do that, he creates algorithms that let satellites produce safe, desired results within time frames needed to complete the mission.
Space flight control has long been a lure for Petersen, ramping up when he was a graduate student in flight dynamics at the University of Michigan in the mid-2010s. Shortly after graduating, he was developing software and determining orbital plans for satellites from the operations floor at the Air Force Research Laboratory in Albuquerque, New Mexico. One of his favorite missions was developing advanced guidance algorithms for Mycroft, an experimental spacecraft launched by the Air Force in 2018 to enhance space object understanding and navigation capabilities. The project also investigated automated control mechanisms used for flight safety and explored methods for enhancing space situational awareness.
Not all of his Air Force work involved fancy equipment and multimillion-dollar spacecraft. In 2013, Petersen was working on a new, efficient algorithm. It needed proving on a test harness but money was tight. Petersen and his co-workers turned to Lego Mindstorms, the small, programmable robotic kits for children. He used the Lego kits to emulate satellite motions — a simple proof of concept that actual satellites could avoid each other in space. That success led to better robots, larger test facilities and more definitive scientific validation.
“I got to say that I played with Legos for the Department of Defense. Who can say that?” Petersen says.
Petersen’s research carries big implications and even bigger goals: A nimble, carefully guided spacecraft that can fix or refuel satellites without humans. Space equipment that’s too large to launch intact can be built in orbit – but only if the satellites doing the assembly can work meters apart and in tandem. Satellites can also benefit from in-flight upgrades to graphics cards or rocket engines. Forty percent of satellites have a failure in their first year of operation. After that, problems become even more routine.
“If we can fix those failures in orbit, the life of a spacecraft can be significantly extended,” Petersen says.
Petersen’s advances in precise guidance and satellite proximity are key to making that happen. That includes evolving robotic arms to better control what happens during satellite docking and repair missions. He’s also looking at ways to increase the life of spacecraft by designing modular “plug and play” components. With this comes another effort focused on making satellites less vulnerable to failure and hacking. That means satellites with multiple parts from different vendors can be operated with fewer concerns about unexpected events.
As satellites get more autonomous, mission-control workers can focus on interpreting incoming data, giving higher-order directives and other human-oriented tasks, Petersen says.
“What I get out of my work is a sense of relief that the overall mission has been accomplished,” he says. “I’m not the most important thing on a satellite team. It’s the science. It’s the mission. If I can make that happen, then that’s the best feeling.”
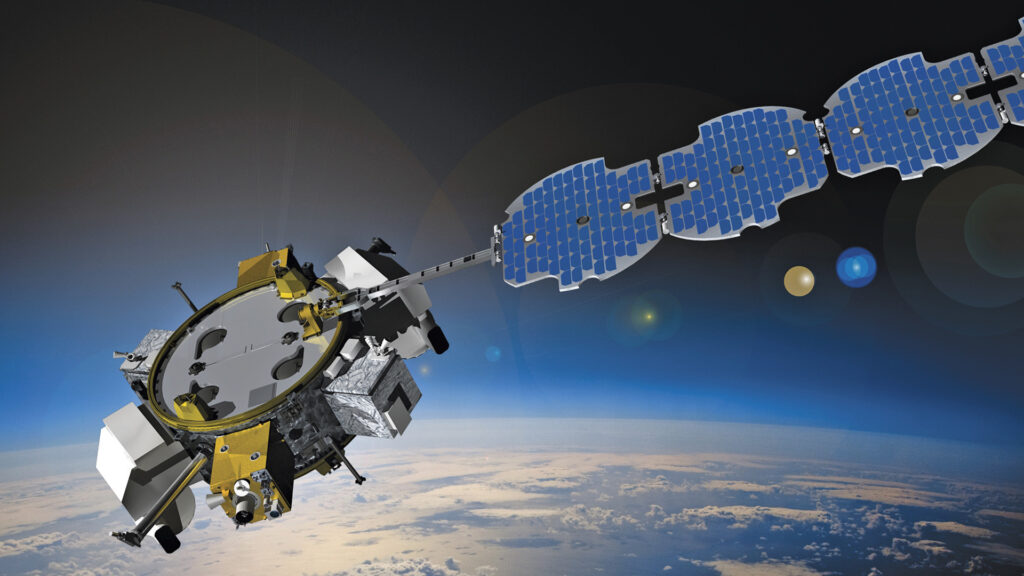
Strengthening Muscle Research
When Siobhan Malany came to Florida in late 2010, space research wasn’t even on her mind. Then, her innate curiosity kicked in.
At the time, Malany was leading a drug discovery team at a non-profit medical research institute in Orlando. When she got a chance to view a space shuttle Endeavour launch, she jumped at the opportunity. That visit helped Malany connect with scientists who were doing crystallography experiments in space. Next came some networking with other researchers through Space Florida, the state’s aerospace economic development agency.
“That was really the introduction to conducting projects in space,” says Malany, an associate professor of pharmacodynamics in the College of Pharmacy.
Her first foray into space research for Space Florida was done on less than a shoestring. Malany convinced some companies to donate materials, then wedged her protein-binding experiment into a 4-inch box that was flown aboard the International Space Station. The experiment showed how a key interaction between vitamins and proteins could be blocked by a drug. That gives researchers the ability to perform drug discovery experiments on common instruments on the ground and in space. By comparing the results, they can determine the effects of microgravity on biological systems.
From that humble beginning, Malany went on to develop a skeletal muscle “lab on a chip” system that was launched to the International Space Station in 2018. Since then, she has sent experiments on three SpaceX resupply missions to the station. The automated cell culturing system is used to study muscle physiology on a human tissue level. Hence, the devices are also called “tissue chips.” The hope is that tissue chips that are grown in three dimensions like real tissue could reduce reliance on animal studies to more accurately model disorders such as sarcopenia, an age-related muscle disease for which there are currently no federally-approved drugs.
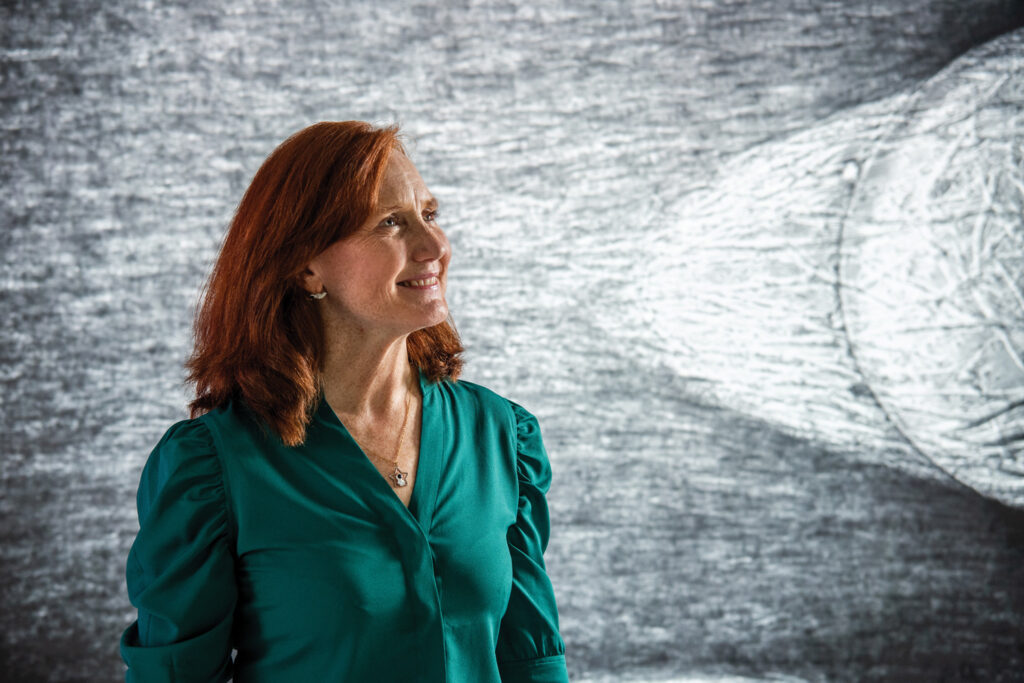
For astronauts, muscle atrophy can be staggering. Studies have shown they lose up to 20% of muscle mass during missions of just two weeks — up to 10 times the amount an early middle-aged person on Earth sheds in a year. Studying muscle cells in space is important for astronauts and earthlings alike, Malany says. Her “lab on a chip” exposes human muscle biopsy cells from younger and older adult donors to all the variables of space flight while being electrically stimulated to mimic exercise.
In 2023, Malany and her collaborators discovered gene expression changes in muscle tissue that was sent into orbit, underscoring the value of space-based tissue research for identifying age-related muscle loss earlier and developing new remedies for sarcopenia. Muscle tissue from younger donors was more sensitive to the space environment and showed greater changes in gene expression compared with older donors, they concluded.
That’s because microgravity speeds up changes to loss of muscle mass and power, giving more information about diseases and aging processes much sooner than terrestrial research. The current cost to treat sarcopenia in the United States is about $40 billion a year.
“That could help us better understand the progression of aging in a shorter period of time — and how to address it on Earth,” Malany says.
On the flip side, astronauts need to live and work in space on longer missions. The “lab on a chip” will be an important platform for studying muscle performance and health in the extreme space environment, she says.
One of her other projects is evaluating whether a compound found in tomato stems and leaves can restore age-related muscle loss like it has been shown to do in studies using mice. In late 2022, 16 samples of skeletal muscle were packed inside Malany’s shoebox-sized, automated CubeLab laboratory. Once aboard the ISS, the muscle cells from the same younger and older people were electrically stimulated and exposed to the tomato compound.
While that analysis is still under way, Malany believes the floating laboratory of space holds promise that goes well beyond muscle cells.
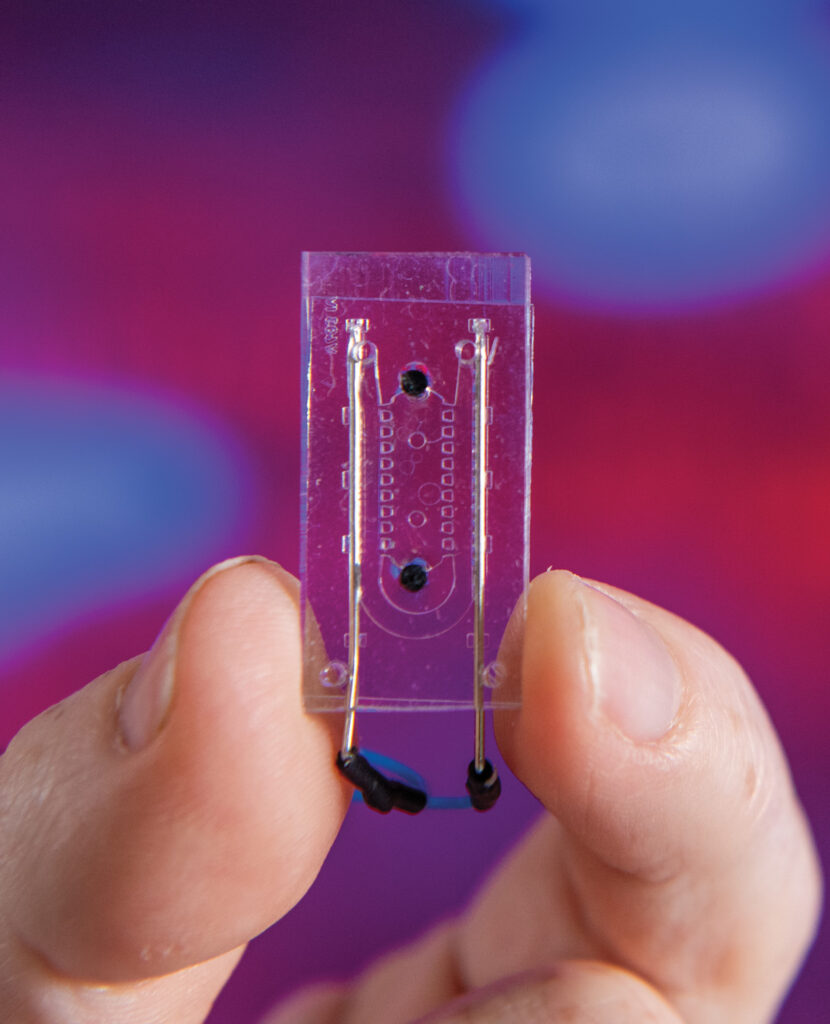
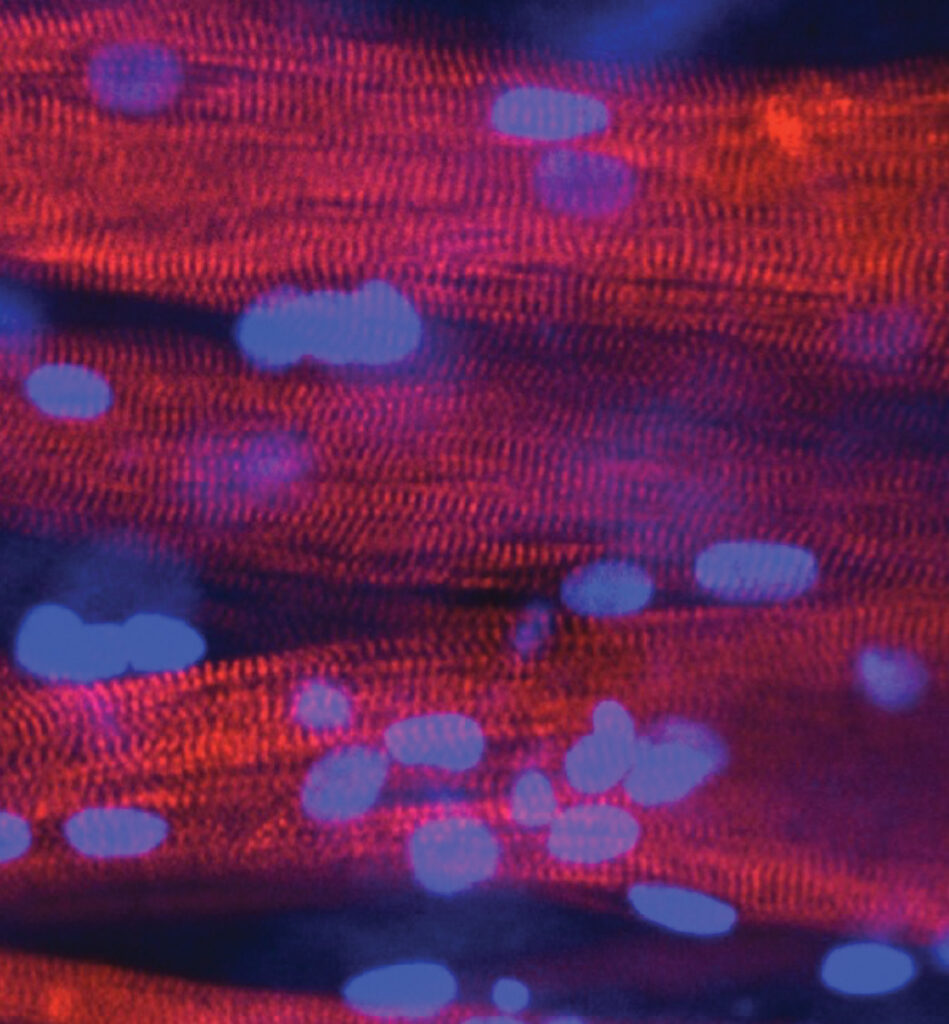
A study assessing astronauts’ liver metabolism was recently funded by UF’s new Astraeus Space Institute and awarded to Maddalena Parafati, a research assistant professor in Malany’s laboratory. Stem cells from astronauts’ blood taken before and after a space mission will be converted into one type of liver cell and analyzed. That approach should provide a trove of data about liver function in space without doing biopsies, Malany says.
Malany also now leads the In-Space Biomanufacturing for Human Health Innovation Hub that was formed late last year through former UF President Ben Sasse’s strategic initiative program. The hub synergizes research in the College of Pharmacy; the departments of Mechanical and Aerospace Engineering, Microbiology and Cell Science, and Biomedical Engineering; the Genetics Institute; and the Space Life Sciences Lab. The intent is to advance in-space biomanufacturing for cell-based products and bioproducts used for preclinical research. It’s particularly exciting, Malany says, because the hub is focusing on shorter-term projects that could have more immediate impacts and potential commercial benefits.
The consortium includes projects studying microgravity’s effect on cardiovascular cell function, which could lead to targeted medications used in space and on Earth. Other research is assessing whether yeast cells can be programmed to make in-flight remedies for astronauts with blood sugar issues and sleep deprivation.
Several faculty are also investigating small particles known as extracellular vesicles, which cancer cells and microbes release naturally to communicate with other cells. If the particles are found to grow and assemble better in space, that might improve their capacity as “nanomedicines” to deliver biological information to cells and tissues.
Those kinds of discoveries are crucial to extending humans’ reach in space, Malany says.
“Mars missions and other long-term explorations are when you need to be producing your own vitamins or the building blocks for other chemicals and drugs,” she says.
Sources:
Siobhan Malany
Associate Professor of Pharmacodynamics
smalany@ufl.edu
Christopher “Chrispy” Petersen
Assistant Professor of Mechanical & Aerospace Engineering
c.petersen1@ufl.edu
Rachael Seidler
Professor of Applied Physiology & Kinesiology
rachaelseidler@ufl.edu