To Edward Bonfiglio, the Avance nerve graft is the difference between keeping his left leg and losing it below the knee to a bullet from an attack in Afghanistan.
To UF biomedical engineer Christine Schmidt, Avance is a biomedical engineering success story.
Commercialized by the University of Florida through Axogen, a company at UF’s Innovation Hub and Sid Martin Biotechnology Incubator, the graft has been used now thousands of times, although Bonfiglio’s case may be the most dramatic.
Avance is an allograft, a cadaver nerve from which cells and tissue have been removed. The remaining structure provides a tunnel to guide the regrowth of peripheral nerves, which, unlike the central nervous system, can regenerate.
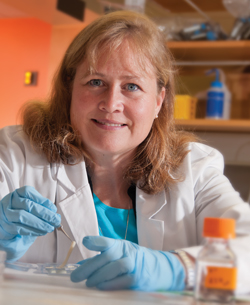
Schmidt says Avance was designed to bridge small gaps between nerves, the kind that might occur when a person cuts a finger in the kitchen. The injury to Bonfiglio’s leg left was much more extensive, but faced with the prospect of amputation, he and his doctors decided to test its limits. And it worked.
“I can walk all day without a problem, even do a light jog,” Bonfiglio says four years later. “I just wanted to keep my leg.”
Schmidt, now chair of UF’s J. Crayton Pruitt Family Department of Biomedical Engineering, developed the decellularization procedure used in the nerve graft with her research group at the University of Texas at Austin. Axogen licensed her technology and combined it with earlier discoveries by David Muir, a UF professor of pediatrics and neuroscience.
Based on her experience working with Axogen, when Schmidt was asked to lead the expanding biomedical engineering department at UF, she was quick to accept.
“This department has everything,” Schmidt says. “At UT, I would have to go to Texas A&M to work with the veterinary school and go to San Antonio to work with the dental school and go to Houston to work with the medical school. Here, everything is co-localized, AND there is a strong innovation component. I know that because my technology didn’t get licensed in Texas, it got licensed here, as part of a UF startup. This is an exciting place to be.”
Founded in 2002, the biomedical engineering department has grown to 16 faculty members, thanks in large part to $10 million in gifts from the late cardiothoracic surgeon J. Crayton Pruitt and his family.
A Magnetic Thread
Jon Dobson, who came to UF from Great Britain, brings expertise in magnetic nanoparticles for use in gene therapy, tissue engineering and regenerative medicine. The high-stakes science and collaborative spirit attracted him.
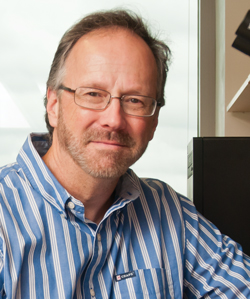
He uses magnetic nanoparticles to deliver DNA and other biomolecules into cells and to target cell surface receptors. The magnetic nanoparticle-targeted receptors can be manipulated via magnetic fields outside the body to activate biochemical signaling pathways that control the cell: for example, telling it to divide, to create tissue matrix, to die.
The magnetic field outside the body acts like a remote control system for activating cell signaling.
“Magnetism is kind of unique in that you can reach inside the body and move things around or deposit energy without actually touching anything,” Dobson says.
Researchers also can heat magnetic nanoparticles, for example in a tumor, making cells more receptive to chemotherapy or killing them. And the particles’ magnetic attraction to each other can be used to induce clustering of molecules to control cell activity. In lab studies, Dobson has taken adult stem cells and used magnetic nanoparticles to control their differentiation into cartilage without using growth factors or chemicals.
The goal is to grow new cartilage, most immediately in a small joint, like a knuckle, but perhaps one day in a knee.
“It’s not that difficult to make cartilage in the lab, but when you put it into the body, is it going to function the way it’s supposed to function? So we’re working on activating a series of surface receptors to not only deposit collagen but deposit the right type of collagen in the right ratio with the right sort of morphology to create functional cartilage,” says Dobson, who started two companies in Great Britain and has a third in mind for osteoarthritis and other therapies.
Controlling cells with magnetic fields has advantages over using chemicals, electrical fields or viruses, Dobson says. Chemicals can be wayward in the body, ending up in non-target cells and staying too long. Electrical fields are an issue because the body conducts electricity, and viruses can be unpredictable.
When Dobson arrived at UF, he noticed more of his colleagues venturing into cell engineering, but struggling with lab space, culturing cells in one lab and hoping they survived the trip to the next lab in 95-degree heat or pouring rain. He suggested a shared facility where anyone with a good idea could work on cells, and found that Dean Cammy Abernathy had been thinking along the same lines. She asked Dobson to lead the Institute for Cell Engineering and Regenerative Medicine, which began operating in the spring.
Collaborations are key, Dobson says. He is working with colleagues in electrical engineering, medicine and materials science on ways to harvest and trap tumor cells, and with another materials science colleague he is working on magnetic actuation to build blood vessels.
Magnetic biomaterials is a growing area. By Dobson’s count, there were less than 1,000 academic journal citations for the topic in 2004; in 2012, there were 12,500.
New Ways to See
Many therapies rely on imaging, and Huabei Jiang, who holds one of three J. Crayton Pruitt Family Professorships, is working on several imaging methods that hold promise.
“One of the major difficulties in diagnosing diseases like cancer is our inability to see inside the body,” Jiang says.
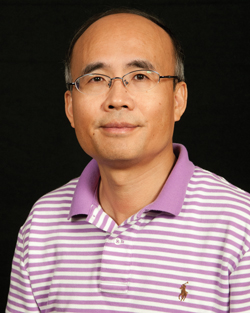
Jiang says photoacoustic imaging is particularly exciting. While an X-ray reveals structural information from inside the body, photoacoustic imaging reveals a three-dimensional image and adds a fourth dimension — time — to provide functional information. Rather than just revealing the existence of a cell, photoacoustic imaging can tell if a cell is alive or dead, whether it has absorbed a drug, whether it has heated up. X-rays can tell the cell is there, but photoacoustic imaging can tell what the cell is doing.
That level of detail could help in treating seizures. Jiang says 80 percent of people with medication-resistant epilepsy could be cured through surgery if the focus of the seizures could be pinpointed quickly and precisely. Other imaging techniques, magnetic resonance imaging, for instance, are too slow to “catch the event and provide a real-time image,” Jiang says.
Breast cancer imaging also could be improved, Jiang believes. In his lab, Jiang has constructed prototype imagers to test optical imaging using light and computing power. A fiber optic laser sends 10 wavelengths, or colors, of light into the breast. The light scatters and is picked up from many directions by detectors, which can reveal clusters of blood vessels that might indicate a tumor. The color also changes depending on chemicals encountered, including chemicals linked to cancer. The size and density of the cells encountered by the light also provide clues to the presence of cancer. Jiang is perfecting the technique, but so far has seen good results in studies.
“MRI is great, but expensive and has relatively low resolution, while X-ray imaging has higher resolution but comes with some dangers. Good, old ultrasound is the happy medium, but you will notice that missing from that list is light,” Jiang says.
Light imaging has great resolution, uses relatively inexpensive equipment and is safe, making it worth investing more in research, Jiang says.
“We want to invent a totally different imaging technology that will really impact medicine,” Jiang says.
“Traditional engineering cannot do this; traditional medicine cannot do this,” Jiang says. “Biomedical engineering is unique, it can do more than the two disciplines alone.”
Radiation in Imaging and Therapy
Almost half the radiation exposure of the U.S. population is from medical sources, according to a 2006 report by the National Council on Radiation Protection and Measurements. Is there cause for concern? Wesley Bolch and his colleagues in the medical physics department, one of only a few in the country housed in a college of engineering rather than physics or medicine, are working on the answer.
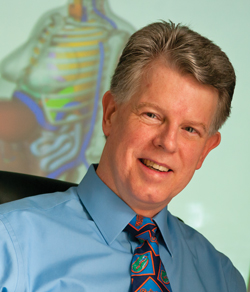
Imaging for diagnosis — CT scans, fluoroscopy, and nuclear medicine — has skyrocketed in recent years. In the 1980s, it took many minutes for X-ray scans, making them rare for small, wiggly children.
“Now you can scan from head to toe in a second or two, very, very, fast,” Bolch says. “Obviously people started scanning children.”
In the 1990s, researchers noticed children were being scanned with adult settings, causing alarm about lifetime radiation exposure from diagnostic imaging. Physicians want the best image, but the real question, Bolch says, should be “what is the minimal quality image produced at the minimum patient dose that still allows the physician to make a diagnosis?”
Complicating matters is that many physicians are unsure about the radiation doses being delivered to their patients. In a recent survey at 20 pediatric hospitals, doctors were asked a hypothetical question: If you had a 7-year-old patient and you wanted to do a low-dose nuclear medicine imaging scan of the kidneys, how much radioactivity — in the form of a radioactive drug — would you give the child? The answers varied by a factor of 20, meaning a child in one hospital could get 20 times the dose as a child in another hospital.
Weight is not a proper guide, either. Two 70-pound children, one tall and skinny, the other short and stout, would require different doses; the tall, skinny child would need half the dose as the short, stout child to produce the same quality image for the diagnosis.
To provide guidance, Bolch and his colleagues in the Advanced Laboratory for Radiation Dosimetry Studies have developed a library of computational phantoms — essentially virtual patients — using the same technology Pixar studio uses for computer animation. The phantoms exist in a database and are three-dimensional computerized models that are detailed and scalable. The UF phantoms, now in their third generation of refinement, have set a world standard in radiation dosimetry and have been adopted as the international standard by the International Commission on Radiological Protection.
A doctor who needs an image can call up a UF phantom — there are 360, so far — that approximates his patient’s gender and build, and the computer calculates the radiation exposure.
“With our phantoms, we can make the dose estimate as close to patient-specific as we can get it,” Bolch says. “A doctor can say, ‘my next patient looks like UF phantom 121, what is the dose?’ With electronic medical records, you could have that recorded for every imaging exam you have.”
Soon, Bolch will add pregnant phantoms, the only ones in the world at this level of fetal organ detail, at gestational ages of 8, 10, 15, 20, 25 and 38 weeks. And for the main reference phantoms, there will be anatomical twins, physical representations of what shows up on the computer screen that will be used to validate dose measurements.
Decade of the Brain II
Stoked by the success of the Human Genome Project — the gene-mapping project that finished years ahead of schedule — President Obama has announced the next big biomedical collaboration: brain activity mapping.
The first Decade of the Brain in the 1990s barely scratched the surface of the research to be done, say UF biomedical engineers like Ranganatha Sitaram.
“This will be a major endeavor of the next decade,” Sitaram says. “I think the brain can learn to change itself.
Sitaram is working on non-invasive methods of collecting brain signals and correlating the signals to function, an emotion for instance, or a grip on a coffee cup. Sitaram’s expertise is in computational intelligence, imaging and neuroscience, and his goal is to decode the signals so that patients can train their brains to make up for a deficit of some kind.
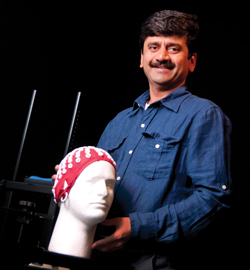
“After the signals are decoded, the information can be used in many ways, for example, to send commands to a computer so that you can control it and do tasks that you would otherwise do with your hand,” a process that would help a person who was paralyzed, Sitaram says.
“Once you have a basic method to transform thinking into action, you can imagine connecting it to a computer and getting that action performed,” he adds.
The applications are many: communication and control for patients paralyzed by stroke or accidents; rehabilitation of psychological disorders, such as schizophrenia; and general scientific investigation of emotion, cognition and motor function.
The next wave of brain research will require more than a map of the brain, however. The connections between the regions — the science of connectomics — will need to be deciphered as well, Sitaram says. The structural and functional connections will be a key to understanding brain-behavior relationships in both healthy brains and abnormal brains.
Sitaram describes himself as an untraditional academic. He worked for 12 years as a research scientist in computation and intelligent algorithms and spun off a company from technology he developed. He was attracted to the opportunity UF offered to work in an interdisciplinary fashion, including with the McKnight Brain Institute, located a short walk from the biomedical sciences building.
“There is excitement in the research here … the ideas you can develop with sheer enthusiasm and hard work. That process changes you as a person,” Sitaram says.
Perhaps that’s why students are beating a path to the department’s doorstep. The first 20 students in the undergraduate biomedical engineering degree program started in 2012, and more than 300 students want in for fall 2013, Schmidt says.
“With strong innovation, and with the medical, dental, veterinary and engineering all here, that makes for wonderful opportunities for translating research, and I’m a big proponent of that,” she adds. “We want to get things into the clinic, impact patients’ lives.”
Sources:
- Christine Schmidt, Professor and Chair, Department of Biomedical Engineering
- Jon Dobson, Professor, Department of Biomedical Engineering
- Huabei Jiang, Professor, Department of Biomedical Engineering
- Wesley Bolch, Professor, Department of Biomedical Engineering
- Ranganatha Sitaram, Assistant Professor, Department of Biomedical Engineering
This article was originally featured in the Summer 2013 issue of Explore Magazine.